Translational Perioperative and Pain Medicine (ISSN: 2330-4871)
ARTICLE DOI: 10.31480/2330-4871/137
Perspective Open Access
Salvinorin A for Stroke Rescue Purposes
John Grothusen, PhD*
Department of Anesthesiology and Critical Care, Perelman School of Medicine at the University of Pennsylvania, Philadelphia, PA 19104, USA
John Grothusen, PhD, Department of Anesthesiology and Critical Care, Perelman School of Medicine at the University of Pennsylvania, 335 John Morgan Building, 3620 Hamilton Walk, Philadelphia, PA 19104, USA, Email: John.Grothusen@pennmedicine.upenn.edu
Editor: Yuan-Xiang Tao, Ph.D., M.D., Professor and Vice Chair, Director, Center for Pain Medicine Research, Department of Anesthesiology, Editor in Chief, The Translational Perioperative and Pain Medicine, Rutgers, The State University of New Jersey, New Jersey Medical School, 185 S. Orange Ave., MSB, E-661, Newark, NJ 07103, USA, Tel: 973-972-9812, E-mail: yt211@njms.rutgers.edu
Received: March 16, 2021 | Accepted: March 25, 2021 | Published: March 26, 2021
Citation: Grothusen J. Salvinorin A for Stroke Rescue Purposes. Transl Perioper & Pain Med 2021; 8(2):337-341.
Abstract
In this perspective, I summarize the current evidence that salvinorin A could be used for stroke rescue. A recent study to demonstrate the effectiveness of intranasal salvinorin A to improve long term neurological outcome in a monkey ischemic stroke model is very promising. Current accumulated evidence indicates that salvinorin A can improve stroke outcome through various mechanisms. While salvinorin A has side effects of diuresis, hypothermia, reduced brain metabolism, and psychotropic effects, these could potentially all contribute to protective effects in the setting of stroke.
Keywords
Salvinorin A, Stroke, Mechanism, Ischemia, Hemorrhagic, Outcome
Introduction
Salvinorin A (SA) is a small molecule (432.4 g/mol) that is the main active component readily isolated from the plant salvia divinorum [1]. It has been ingested for centuries for religious ritual and recreational purposes [2]. SA is a kappa opioid receptor (KOR) agonist that isthe most potent KOR agonist from a natural source known to date. It displays no respiratory depression, and there is limited or no addiction potential [3] reported so far despite decades of human usage. It is unique in that it is a non-opioid molecule structurally unrelated to any other known opioid agonists. It is a hydrophobic molecule that rapidly enters the brainand that can reach peak concentration within seconds [4,5]. We have discussed its potential usage for stroke therapy [6]. A recent landmark study demonstrated the effectiveness of SA in improving long term neurological outcome (28 days after stroke) in a monkey stroke model [7]. The critical importance of this study is that it used a monkey model with autologous blood clot to closely simulate the common clinical stroke scenario. The decrease of the infarct size and improvement in long term neurological outcome is encouraging and convincing, despite being a small scale preliminary trial. This study used an intranasal SA administration strategy for potential acute rescue purposes. An intranasal formulation allows for easy and rapid delivery of SA in emergent and pre-hospital settings. This route also avoids the hepatic first-pass metabolism [8] and direct hydrolysis of SA from intravenous administration. In addition, intranasal administration of appropriately formulated drugs can directly access the brain by bypassing the blood brain barrier and reducing off-target effects [9]. It is important to point out also that other potential neuroprotective agents are generally administered intravenously. In a stroke, the problem is lack of blood flow to a region of the brain, therefore an intravenous therapy would be severely limited in its access to the part of the brain that needs protection the most. An intranasal approach with a small hydrophobic molecule like SA can access all areas of the brain by simple diffusion.
The Evidence of Protective Effects in Various Preclinical Models
The first study of SA on the brain neurovascular unit started with the discovery that SA can selectively dilate brain vessels via the KOR receptor and KATP channels and nitric oxide pathway in a piglet model [10]. Subsequently, it was discovered that SA can preserve cerebral vascular autoregulation in a piglet model of hypoxia/ischemia whether it is given prior to ischemia or after reperfusion [11,12]. SA reduces neonatal mortality and improves neurological outcome in a rodent neonatal hypoxia model. Intranasal SA improves neurological outcome in a rodent middle cerebral artery occlusion (MCAO) model [13]. One of the major concerns with any potential stroke rescue therapeutic is whether a drug like SA with known cerebral vasodilatation properties [10] would be contraindicated in hemorrhagic stroke, or if it could cause or exacerbate hemorrhagic transformation of an ischemic stroke. Hemorrhagic transformation, hemorrhagic infarction and parenchymal hematoma, following ischemic stroke is a significant concern, being the result of multiple co-existing factors both systemic and cerebral [14]. Major contributors to hemorrhagic transformation of ischemic stroke are the size of the infarct and reduction of collateral perfusion of the surrounding penumbra [15] and loss of cerebral blood flow autoregulation [16]. SA has been shown to reduce ischemic infarct size [7,13], and along with its vasodilatory properties [10] and protection of cerebral autoregulation [11] would be expected to potentially reduce the possibility of hemorrhagic transformation of ischemic stroke. Current evidence also indicates that SA has robust efficacy in a model of hemorrhagic stroke, the mouse subarachnoid hemorrhage model [17]. Efficacy, or at a minimum no additional harm, in hemorrhagic stroke is of critical importance to any new acute stroke therapeutic. Current treatment, specifically tPA requires a neuroimaging study be performed prior to administration, since clot dissolution would definitely be contraindicated in hemorrhagic stroke. A drug that would benefit hemorrhagic stroke, or at least not exacerbate it, would save precious time by being able to be administered immediately after recognition of stroke, and prior to precious time-consuming definitive hospital based neurodiagnostic confirmation of the type of stroke. Neuroprotective effects of other KOR agonists besides SA have been demonstrated in brain hypoxia and ischemia models in a variety of different animal species [18], but so far none, other than SA, have demonstrated significant clinical value due to their intrinsic opioid characteristics, low selectivity, or lack of clinical safety profiles [19]. Many attempts at developing acute stroke therapeutics have been tried and ultimately failed to deliver on clinically significant neuroprotection. KOR agonists, specifically SA, are fundamentally different in action from that of many other potential therapeutics based on specific targets such as those posited by the 'excitotoxicity' model of stroke [20,21]. KOR agonists, especially SA, need to be developed further and advanced to clinical trials as soon as possible.
Mechanism of Actions
It is clear that the key mechanism of SA protective effects is through KOR activation. Use of a KOR antagonist can abolish the protective effects and the brain vascular dilatation effects of SA [10,13]. Based on a recently published study using a cell culture hypoxia model, it appears that SA may be working through the beta-arrestin pathway rather than through the G protein pathway [22]. KORs are widely expressed throughout the brain. Evidence that KORs redistribute during ischemia [13,23], and data on the phenomenon of pre- and post-conditioning of ischemic stress [24] indicate that opioid receptors are functionally part of a natural defensive response to a sudden lack of blood flow [23]. This idea is borne out by the pleiotropic nature of the effects of SA in cerebral ischemia studies. SA has been shown to possess selective cerebral vessel dilatation properties [10], anti-inflammatory properties [17,25], cerebral auto-regulation protection [11], immuno-modulation properties [26], and diuretic effects [19]. Of major significance is that SA has been shown to protect the blood brain barrier in ischemia [13]. Another potentially important effect of KOR agonists, including SA, is hypothermia [27,28]. KOR mediated hypothermia has been shown to reduce metabolic demand during caloric restriction [29]. Cerebral hypothermia may play a role in neuroprotection, as it has been reported that hippocampal damage is almost completely abolished in rats by a reduction of only 2-3 °C in the brain during ischemia [30,31]. It is also possible that SA could offer protective effects through immune-modulation, based on a recent study on pulmonary macrophages and the inflammation process [32,33]. Table 1 summarizes the multiple potential mechanisms and evidence that SA could offer an exciting new therapeutic option in the setting of acute stroke rescue based on the current available experimental data.
Table 1: Why Salvinorin A is suitable for stroke therapy.
Selective brain vessel dilatation |
[10] |
Anti-inflammatory properties |
|
Cerebral auto-regulation protection |
[11] |
Immuno-modulation |
[26] |
Protects blood brain barrier |
[13] |
Diuretic effects |
[19] |
Small molecule |
[37] |
Passes through blood brain barrier |
[4] |
Easy administration (intranasal etc.) |
[38] |
Quick onset (within seconds) |
[39] |
High potency (nM) |
[37] |
Hypothermic effects |
[40] |
Decrease brain metabolism |
[5] |
Acts on specific receptor |
[41] |
History of long-term human use |
[2] |
From natural source |
[1] |
Mouse stroke model efficacy |
[13] |
Pig hypoxia model efficacy |
|
Monkey stroke model efficacy |
[7] |
Brain ischemia model efficacy |
[13] |
Subarachnoid hemorrhage efficacy |
[17] |
An example of our ongoing efforts to study potential direct neuronal protective effects of SA is shown in Figure 1 (unpublished data). For this study rat primary culture cortical neurons were exposed to oxygen and glucose deprivation to ascertain whether SA has can preserve cellular structural features observed under electron microscopy. As indicated in the figure, our preliminary results indicate that subcellular structure appears to be protected in rat primary cortical neuronal cells exposed to SA during 4 hours of oxygen glucose deprivation (OGD).
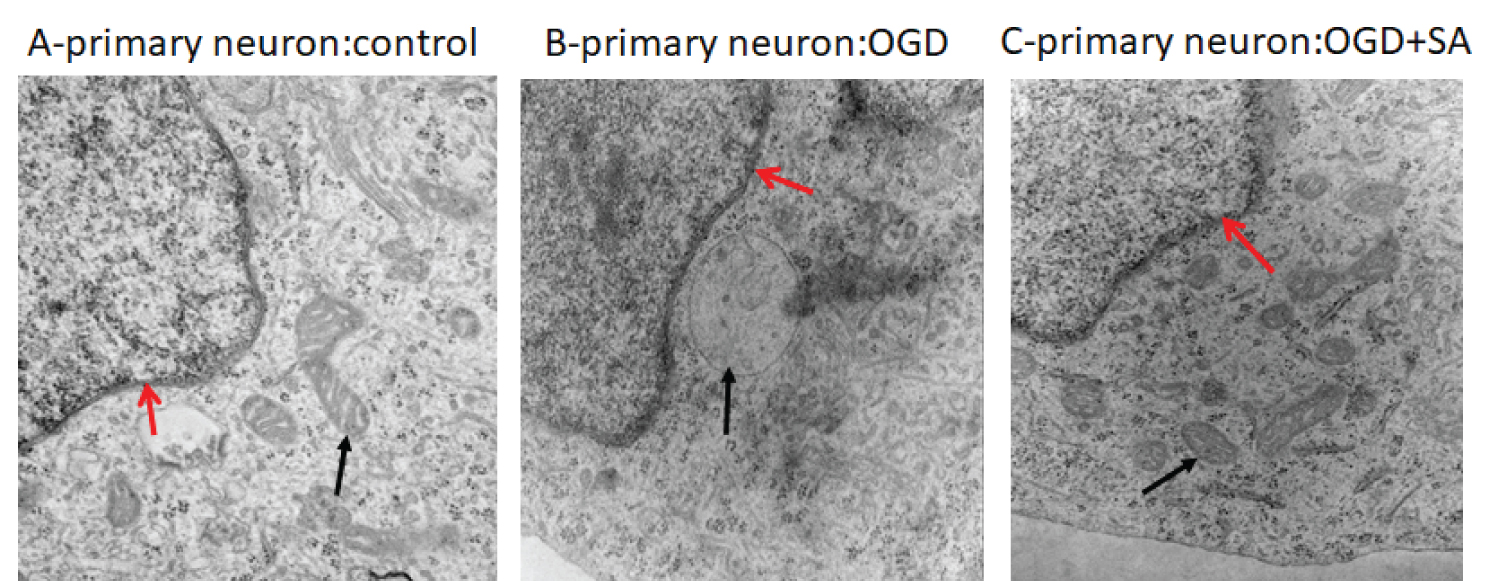
Figure 1: Subcellular changes in the rat primary cortical neuron. (A) Nuclear (red arrow) and well-structured mitochondria (black arrow) are seen in control; (B) Swollen mitochondria are observed in OGD exposed cortical neurons (black arrow); (C) Morphology of mitochondria are preserved by SA (10 μM) under OGD (black arrow). OGD: Oxygen glucose deprivation. EM direct magnification: 50,000 (author's unpublished data).
Psychotropic Effect of SA
SA has been reported to have rapid and short-lived euphoric hallucinogenic activity, rather than the dysphoric effects seen in classic KOR agonists [34]. In human studies, no persisting adverse effects related to SA were observed [34]. Rapid onset and transient psychoactive activity indicates that the drug does in fact get into the brain very quickly and has an effect on neural function, which is exactly what a fast acting pleiotropic rescue medication should do. Psychotropic agents may, in fact, be neuroprotective [35] and there is some evidence that drug induced euphoria is associated with reduced cerebral metabolism [36]. There is, as far as we know, no a priori reason to assume that the rapid hallucinogenic and euphoric activity is separable from the rapid pleiotropic neuroprotective effects of SA.
Future Research Direction
SA has been shown to possess attributes that make it a very exciting potential stroke rescue therapy. Studies are needed to address the timing of the dosing to achieve significant potential clinical benefit. In particular, how long from onset of stroke can SA be given and still provide significant reduction of infarct and subsequent improvement of stroke outcome? A formulation of SA, specifically for intranasal administration, that is stable and can be directly translated from pre-clinical to clinical use is also needed.
In summary, current evidences indicated that salvinorin A could be a critical game changer in stroke rescue if it can be developed and advanced into clinical practice. The psychoactive effects should not be the huge hurdle it's been perceived as to block the development of this potential life-saving and disability preventing acute rescue strategy for stroke patients.
Conflict of Interests
None.
Funding
Department of Anesthesiology and Critical Care, Perelman School of Medicine at the University of Pennsylvania.
References
- Roth BL, Baner K, Westkaemper R, Siebert D, Rice KC, Steinberg S, et al. Salvinorin A: A potent naturally occurring nonnitrogenous kappa opioid selective agonist. Proc Natl Acad Sci U S A. 2002;99(18):11934-9. Epub 2002/08/23. doi: 10.1073/pnas.182234399182234399 [pii]. PubMed PMID: 12192085; PubMed Central PMCID: PMC129372.
- Hernandez-Bello R, Garcia-Rodriguez RV, Garcia-Sosa K, Pena-Rodriguez LM, Vazquez-Hernandez M, Ramos-Morales FR, et al. Salvinorin A content in legal high products of Salvia divinorum sold in Mexico. Forensic Sci Int. 2015;249:197-201. Epub 2015/02/25. doi: 10.1016/j.forsciint.2015.01.038. PubMed PMID: 25710779.
- Kivell BM, Ewald AW, Prisinzano TE. Salvinorin A analogs and other kappa-opioid receptor compounds as treatments for cocaine abuse. Adv Pharmacol. 2014;69:481-511. Epub 2014/02/04. doi: 10.1016/B978-0-12-420118-7.00012-3. PubMed PMID: 24484985; PubMed Central PMCID: PMCPMC4128345.
- Hooker JM, Xu Y, Schiffer W, Shea C, Carter P, Fowler JS. Pharmacokinetics of the potent hallucinogen, salvinorin A in primates parallels the rapid onset and short duration of effects in humans. Neuroimage. 2008;41(3):1044-50. Epub 2008/04/25. doi: 10.1016/j.neuroimage.2008.03.003. PubMed PMID: 18434204; PubMed Central PMCID: PMCPMC2464626.
- Hooker JM, Patel V, Kothari S, Schiffer WK. Metabolic changes in the rodent brain after acute administration of salvinorin A. Mol Imaging Biol. 2009;11(3):137-43. Epub 2009/01/10. doi: 10.1007/s11307-008-0192-x. PubMed PMID: 19132449; PubMed Central PMCID: PMC2744594.
- Grothusen J, Liu R. Salvinorin A. As a Potential Medication for Stroke. Transl Perioper Pain Med. 2019;6(4):115-9. doi: DOI: 10.31480/2330-4871/098.
- Wu L, Wu D, Chen J, Chen C, Yao T, He X, et al. Intranasal salvinorin A improves neurological outcome in rhesus monkey ischemic stroke model using autologous blood clot. J Cereb Blood Flow Metab. 2020:271678X20938137. Epub 2020/07/04. doi: 10.1177/0271678X20938137. PubMed PMID: 32615886.
- Dhuria SV, Hanson LR, Frey WH, 2nd. Intranasal delivery to the central nervous system: Mechanisms and experimental considerations. J Pharm Sci. 2010;99(4):1654-73. Epub 2009/10/31. doi: 10.1002/jps.21924. PubMed PMID: 19877171.
- Zhu J, Jiang Y, Xu G, Liu X. Intranasal administration: A potential solution for cross-BBB delivering neurotrophic factors. Histology and histopathology. 2012;27(5):537-48. Epub 2012/03/16. PubMed PMID: 22419018.
- Su D, Riley J, Kiessling WJ, Armstead WM, Liu R. Salvinorin A produces cerebrovasodilation through activation of nitric oxide synthase, kappa receptor, and adenosine triphosphate-sensitive potassium channel. Anesthesiology. 2011;114(2):374-9. PubMed PMID: 21245734.
- Su D, Riley J, Kiessling WJ, Armstead WM, Liu R. Salvinorin A pretreatment preserves cerebrovascular autoregulation after brain hypoxic/ischemic injury via ERK/MAPK in piglets. Anesth Analg. 2012;114(1):200-4. Epub 2011 Nov 10.; PubMed Central PMCID: PMCnot available yet.
- Wang Z, Ma N, Riley J, Armstead WM, Liu R. Salvinorin A administration after global cerebral hypoxia/ischemia preserves cerebrovascular autoregulation via kappa opioid receptor in piglets PloS ONE. 2012;7(7):e41724.
- Chen C, Xi C, Liang X, Ma J, Su D, Abel T, et al. The Role of kappa Opioid Receptor in Brain Ischemia. Crit Care Med. 2016;44(12):e1219-e25. doi: 10.1097/CCM.0000000000001959. PubMed PMID: 27495821; PubMed Central PMCID: PMCPMC5203937.
- Pande SD, Win MM, Khine AA, Zaw EM, Manoharraj N, Lolong L, et al. Haemorrhagic transformation following ischaemic stroke: A retrospective study. Sci Rep. 2020;10(1):5319. Epub 2020/03/27. doi: 10.1038/s41598-020-62230-5. PubMed PMID: 32210323; PubMed Central PMCID: PMCPMC7093519.
- Zhang J, Yang Y, Sun H, Xing Y. Hemorrhagic transformation after cerebral infarction: current concepts and challenges. Ann Transl Med. 2014;2(8):81. Epub 2014/10/22. doi: 10.3978/j.issn.2305-5839.2014.08.08. PubMed PMID: 25333056; PubMed Central PMCID: PMCPMC4200641.
- Silverman A, Kodali S, Sheth KN, Petersen NH. Hemodynamics and Hemorrhagic Transformation After Endovascular Therapy for Ischemic Stroke. Front Neurol. 2020;11:728. Epub 2020/08/09. doi: 10.3389/fneur.2020.00728. PubMed PMID: 32765416; PubMed Central PMCID: PMCPMC7379334.
- Sun J, Yang X, Zhang Y, Zhang W, Lu J, Hu Q, et al. Salvinorin A attenuates early brain injury through PI3K/Akt pathway after subarachnoid hemorrhage in rat. Brain research. 2019;1719:64-70. Epub 2019/05/28. doi: 10.1016/j.brainres.2019.05.026. PubMed PMID: 31125530.
- Goyagi T, Toung TJ, Kirsch JR, Traystman RJ, Koehler RC, Hurn PD, et al. Neuroprotective kappa-opioid receptor agonist BRL 52537 attenuates ischemia-evoked nitric oxide production in vivo in rats. Stroke. 2003;34(6):1533-8. Epub 2003/05/10. doi: 10.1161/01.STR.0000072512.30658.E7. PubMed PMID: 12738895.
- Chen C, Xi C, Sujita M, Liu R. Kappa Opioid Receptor Agonist and Brain Ischemia. Transl Perioper Pain Med. 2014;1(2):27-34. Epub 2015/01/13. PubMed PMID: 25574482; PubMed Central PMCID: PMCPMC4285354.
- Lai TW, Zhang S, Wang YT. Excitotoxicity and stroke: identifying novel targets for neuroprotection. Prog Neurobiol. 2014;115:157-88. Epub 2013/12/24. doi: 10.1016/j.pneurobio.2013.11.006. PubMed PMID: 24361499.
- Shi L, Rocha M, Leak RK, Zhao J, Bhatia TN, Mu H, et al. A new era for stroke therapy: Integrating neurovascular protection with optimal reperfusion. J Cereb Blood Flow Metab. 2018;38(12):2073-91. Epub 2018/09/08. doi: 10.1177/0271678X18798162. PubMed PMID: 30191760; PubMed Central PMCID: PMCPMC6282224.
- Xu J, Chen F, Wang S, Akins NS, Hossain MI, Zhou Y, et al. Kappa opioid receptors internalization is protective against oxygen-glucose deprivation through beta-arrestin activation and Akt-mediated signaling pathway. Neurochem Int. 2020;137:104748. Epub 2020/04/28. doi: 10.1016/j.neuint.2020.104748. PubMed PMID: 32339667; PubMed Central PMCID: PMC7321825.
- Xi C, Liang X, Chen C, Babazada H, Li T, Liu R. Hypoxia induces internalization of kappa-opioid receptor. Anesthesiology. 2017;126(5):842-54. doi: 10.1097/ALN.0000000000001571. PubMed PMID: 28230651; PubMed Central PMCID: PMCPMC5395330.
- Headrick JP, See Hoe LE, Du Toit EF, Peart JN. Opioid receptors and cardioprotection - 'opioidergic conditioning' of the heart. Br J Pharmacol. 2015;172(8):2026-50. Epub 2014/12/19. doi: 10.1111/bph.13042. PubMed PMID: 25521834; PubMed Central PMCID: PMCPMC4386979.
- Aviello G, Borrelli F, Guida F, Romano B, Lewellyn K, De Chiaro M, et al. Ultrapotent effects of salvinorin A, a hallucinogenic compound from Salvia divinorum, on LPS-stimulated murine macrophages and its anti-inflammatory action in vivo. J Mol Med (Berl). 2011;89(9):891-902. Epub 2011/04/19. doi: 10.1007/s00109-011-0752-4. PubMed PMID: 21499737.
- Xu J, Wei K, Zhang G, Lei L, Yang D, Wang W, et al. Ethnopharmacology, phytochemistry, and pharmacology of Chinese Salvia species: A review. J Ethnopharmacol. 2018;225:18-30. Epub 2018/06/24. doi: 10.1016/j.jep.2018.06.029. PubMed PMID: 29935346.
- Handler CM, Geller EB, Adler MW. Effect of mu-, kappa-, and delta-selective opioid agonists on thermoregulation in the rat. Pharmacol Biochem Behav. 1992;43(4):1209-16. Epub 1992/12/01. doi: 10.1016/0091-3057(92)90504-9. PubMed PMID: 1361992.
- Ansonoff MA, Zhang J, Czyzyk T, Rothman RB, Stewart J, Xu H, et al. Antinociceptive and hypothermic effects of Salvinorin A are abolished in a novel strain of kappa-opioid receptor-1 knockout mice. J Pharmacol Exp Ther. 2006;318(2):641-8. Epub 2006/05/05. doi: 10.1124/jpet.106.101998. PubMed PMID: 16672569.
- Cintron-Colon R, Johnson CW, Montenegro-Burke JR, Guijas C, Faulhaber L, Sanchez-Alavez M, et al. Activation of Kappa opioid receptor regulates the hypothermic response to calorie restriction and limits body weight loss. Curr Biol. 2019;29(24):4291-9 e4. Epub 2019/12/02. doi: 10.1016/j.cub.2019.10.027. PubMed PMID: 31786059; PubMed Central PMCID: PMCPMC6917995.
- Sakai H, Sheng H, Yates RB, Ishida K, Pearlstein RD, Warner DS. Isoflurane provides long-term protection against focal cerebral ischemia in the rat. Anesthesiology. 2007;106(1):92-9; discussion 8-10. Epub 2007/01/02. doi: 10.1097/00000542-200701000-00017. PubMed PMID: 17197850.
- Busto R, Dietrich WD, Globus MY, Valdes I, Scheinberg P, Ginsberg MD. Small differences in intraischemic brain temperature critically determine the extent of ischemic neuronal injury. J Cereb Blood Flow Metab. 1987;7(6):729-38. Epub 1987/12/01. doi: 10.1038/jcbfm.1987.127. PubMed PMID: 3693428.
- Zeng S, Chen D, Liu G, Wu YX, Gao ZQ, Su Y, et al. Salvinorin A protects against methicillin resistant staphylococcus aureus-induced acute lung injury via Nrf2 pathway. Int Immunopharmacol. 2021;90:107221. Epub 2020/12/10. doi: 10.1016/j.intimp.2020.107221. PubMed PMID: 33293260.
- Zeng S, Zhong Y, Xiao J, Ji J, Xi J, Wei X, et al. Kappa opioid receptor on pulmonary macrophages and immune function. Translational perioperative and pain medicine. 2020;7(3):225-33. Epub 2020/11/19. doi: 10.31480/2330-4871/117. PubMed PMID: 33204767; PubMed Central PMCID: PMC7668421.
- Johnson MW, MacLean KA, Reissig CJ, Prisinzano TE, Griffiths RR. Human psychopharmacology and dose-effects of salvinorin A, a kappa opioid agonist hallucinogen present in the plant Salvia divinorum. Drug Alcohol Depend. 2011;115(1-2):150-5. doi: 10.1016/j.drugalcdep.2010.11.005. PubMed PMID: 21131142; PubMed Central PMCID: PMCPMC3089685.
- Hunsberger J AD, Henter I, Chen G. The neurotrophic and neuroprotective effects of psychotropic agents. Dialogues in Clinical Neuroscience 2009;11:333-48.
- London ED, Cascella NG, Wong DF, Phillips RL, Dannals RF, Links JM, et al. Cocaine-induced reduction of glucose utilization in human brain. A study using positron emission tomography and [fluorine 18]-fluorodeoxyglucose. Arch Gen Psychiatry. 1990;47(6):567-74. Epub 1990/06/01. doi: 10.1001/archpsyc.1990.01810180067010. PubMed PMID: 2350209.
- Orton E, Liu R. Salvinorin A: A mini review of physical and chemical properties affecting its translation from research to clinical applications in humans. Transl Periop & Pain Med. 2014;1(1):9-11.
- Chen C, Zhang M, Wu Y, Zhou C, Liu R. Intranasal delivering method in the treatment of ischemic stroke. Springer; 2019. p. 75-89.
- Addy PH. Acute and post-acute behavioral and psychological effects of salvinorin A in humans. Psychopharmacology (Berl). 2012;220(1):195-204. Epub 2011/09/09. doi: 10.1007/s00213-011-2470-6. PubMed PMID: 21901316.
- Ansonoff MA, Zhang J, Czyzyk T, Rothman RB, Stewart J, Xu H, et al. Antinociceptive and hypothermic effects of Salvinorin A are abolished in a novel strain of kappa-opioid receptor-1 knockout mice. The Journal of pharmacology and experimental therapeutics. 2006;318(2):641-8. Epub 2006/05/05. doi: 10.1124/jpet.106.101998. PubMed PMID: 16672569.
- Yan F, Roth BL. Salvinorin A: a novel and highly selective kappa-opioid receptor agonist. Life Sci. 2004;75(22):2615-9. Epub 2004/09/17. doi: 10.1016/j.lfs.2004.07.008. PubMed PMID: 15369697.
- Correction to: Accuracy of Prediction Instruments for Diagnosing Large Vessel Occlusion in Individuals With Suspected Stroke: A Systematic Review for the 2018 Guidelines for the Early Management of Patients With Acute Ischemic Stroke. Stroke. 2018;49(3):e139. Epub 2018/02/28. doi: 10.1161/STR.0000000000000165. PubMed PMID: 29483363.