Translational Perioperative and Pain Medicine (ISSN: 2330-4871)
ARTICLE DOI: 10.31480/2330-4871/098
Mini reviewOPEN ACCESS
Salvinorin A as a Potential Medication for Stroke
John Grothusen, PhD and Renyu Liu, MD, PhD*
Department of Anesthesiology and Critical Care, Perelman School of Medicine, University of Pennsylvania, Philadelphia, Pennsylvania, USA
Renyu Liu, MD, PhD, Associate professor, Department of Anesthesiology and Critical Care, Perelman School of Medicine, University of Pennsylvania, 336 John Morgan Building, 3620 Hamilton Walk, Philadelphia, Pennsylvania 19104, USA, E-mail: RenYu.Liu@pennmedicine.upenn.edu.
Editor: Henry Liu, MD, MS, FASA, Professor of Anesthesiology, Vice Chairman for Research, Drexel University College of Medicine, Hahnemann University Hospital, 245 N. 15th Street, MS 310, Philadelphia, PA 19102, USA, Email: henryliupa@gmail.com
Received: August 19, 2019 | Accepted: August 21, 2019| Published: August 22, 2019
Citation: Grothusen J, Liu R. Salvinorin A as a Potential Medication for Stroke. Transl Perioper & Pain Med 2019; 6 (4):115-119.
Abstract
This article is an overview of our effortstowards developing salvinorin A, which is a non-opioid kappa opioid receptor (KOR) agonist and an active component from the plant Salvia divinorum, for clinical usein the treatment of acute stroke. The intrinsic characteristics of salvinorin A, which include rapid onset of action, easy passage through the blood brain barrier with intranasal administration, high potency in regards to neurological protection and awell-documented mechanism of action involving KOR agonism, makes it a promising potential therapeutic agent for acute stroke. Further studies are warranted to develop this compound for clinical use.
Introduction
Stroke, including ischemic and hemorrhagic stroke, is one of the leading causes of death and life-long disability worldwide, despite recent advances in thrombolytic therapy and mechanical thrombectomy [1,2]. Scientists, clinicians and pharmaceutical companies have devoted enormous efforts in developing novel medications for stroke therapy. Many potential neuro-protectants have been tested in animal models with varying levels of success. However, none have been able to achieve significant success in clinical trials [3,44]. As of August 17, 2019, searching "stroke" as a key word in PubMed (https://www.ncbi.nlm.nih.gov/pubmed ) yielded 320948 results and searching "neuroprotection" as a keyword yielded 135700 results. For a potential neuro-protectant to be used in clinical practice for stroke, especially in the acute phase, we believe that it has to fulfil the following key requirements: 1) It has to be potent enough to generate a neuroprotective effect; 2) It has to easily pass the blood brain barrier (BBB); 3) It can be delivered easily to the patient in a timely manner; 4) It is safe and has minimal side effects; 5) It has to target the mechanisms that are clearly related to brain ischemia. It would be ideal if the potential compound could be used in both ischemic and hemorrhagic stroke in the acute phase, thereby eliminating the loss of valuable time waiting for CT scan image confirmation of the stroke type. In an effort to seek potential compounds for stroke treatment, we found that salvinorin A (SA) is a highly hopeful compound as discussed below.
What is SA?
SA is a non-opioid, highly selective Kappa Opioid Receptor (KOR) agonist. It is an active component from Salvia divinorum which is a perennial herb that has been consumed by humans for recreational and religious ritual purposes for several centuries [5]. Thus, we could say that in effect, SA has undergone lengthy testing in human populations. Unlike other KOR agonists, SA produces no frank hallucinatory or dysphoric effects. In a clinical trial in healthy human subjects, no persisting adverse effects related to SA were observed [6]. Due to the existence of an ester linkage in the structure of SA, and numerous esterase enzymes in the body, the pharmacological effects of SA are short-lived. Traditional opioid ligands have nitrogen in their structure, and since there is no nitrogen in the molecule of SA, it is considered as the first non-nitrogenous or non-opioid KOR agonist. Our initial interest in SA started from its unique molecular structure and potential pharmacological effects, stemming from our experience in the perioperative arena seeking novel medications with quick onset and offset characteristics.
A Potent Cerebral Vascular Dilator
Using a piglet model with a surgically installed transparent cranial window, we were surprised to have noticed that SA is a very potent cerebral vascular dilator. As seen in Figure 1, we found that upon addition of SA, a normal cerebral artery on the surface of the piglet brain became dilated within seconds of SA administration (Figure 1).
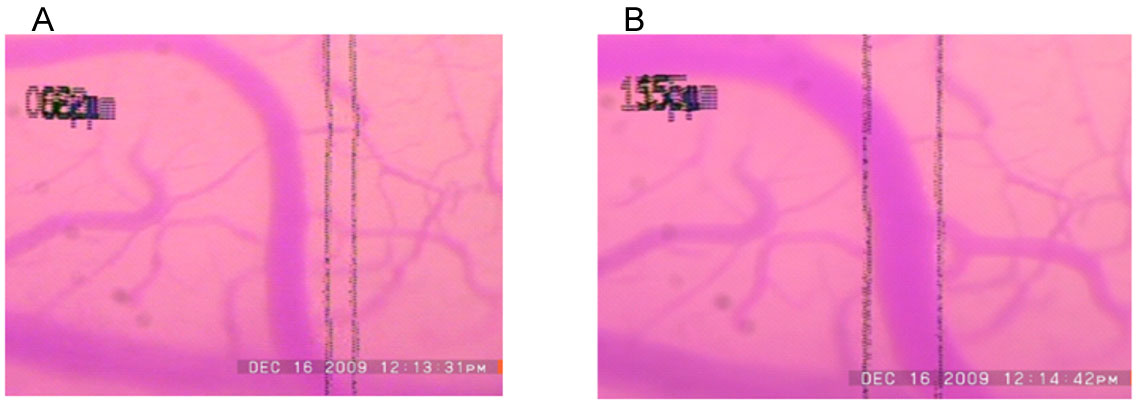
Figure 1: Original images of the pial artery of a piglet in the absence (image A) and presence of SA (image B). The small arteries on the surface of the piglet brain were imaged using a microscope. The diameters of the vessels can be measured using a calibrated scale. Image A on the is the baseline image of the small arteries on the surface of the brain. Image B is the image on the same microscopic field taken one minute after administration of SA. As noted, the diameter of the small vessels increased significantly, which would potentially improve collateral circulation also. SA, Salvinorin A.
Interestingly, there was no significant change in systemic blood pressure with SA administration. We demonstrated that SA not only can dilate the pial artery under normal conditions, but also under the vasoconstricted conditions induced by hypocarbia and endothelin, indicating that SA could be used to dilate brain vessels in pathological conditions [7]. Such cerebral vascular dilation effects have been proven to be through KOR activation since such effects can be blocked by a non-specific (naloxone) and specific KOR antagonist (norbinaltorphimine) [7].
The Effects on Brain Ischemia
Inspired by the discovery of selective cerebral vascular dilative effects, we advanced our efforts to test whether SA could protect the brain from hypoxia and ischemia by using both piglet and rodent brain ischemia models. As we expected, the administration of SA before, and more importantly, after brain hypoxia and ischemia, protected the cerebral vascular auto-regulation significantly in the piglet model [8,9]. As shown in Figure 2, brain arterial autoregulation is abolished by brain ischemia. SA treatment preserved autoregulation and a KOR antagonist blocked the protective effects of SA on vascular autoregulation [8].
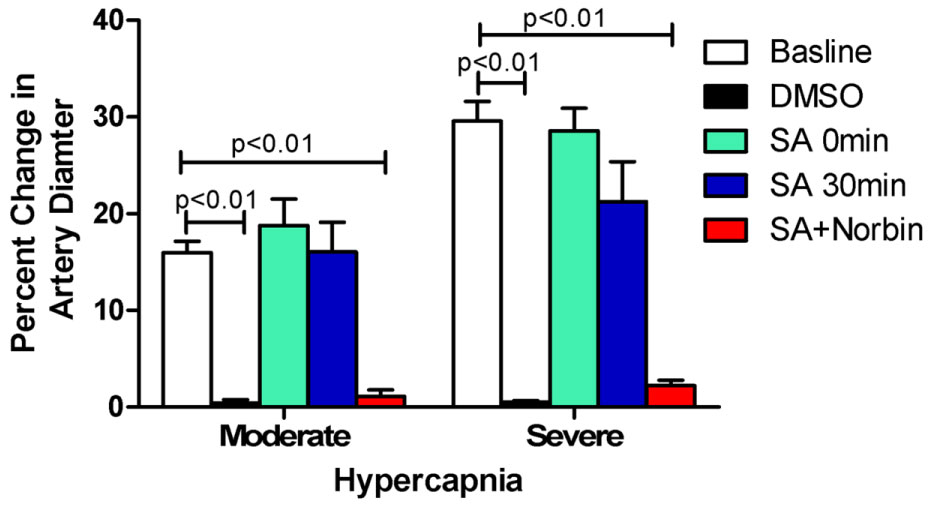
Figure 2: Autoregulation of the cerebral artery. In the normal condition at baseline, the diameter of the artery increases with increase of CO2 in the blood induced by hypercapnia, a protective mechanism of autoregulation to ensure the brain receives more blood in the presence of potential hypoxia. This protective mechanism of increased diameter with hypercapnia is abolished following brain hypoxia and ischemia as seen in the vehicle only control (DMSO); but preserved well in the presence of SA (in DMSO) given immediately and 30 min of after reperfusion following hypoxia and ischemia. The specific kappa opioid receptor antagonist, norbinaltorphimine (Norbin), abolished such protective effects. N = 5 in each group. Percentage change = (diameter after hypercapnia−diameter before hypercapnia)/diameter before hypercapnia) *100. SA: Salvinorin A; Moderate: hypercapnia with PaCO2 of 50 to 60 mmHg; Severe: hypercapnia with PaCO2 of 70 to 80 mmHg. Please refer to the original article for this figure (Wang Z, et al. PloS ONE. 2012;7(7):e41724).[8] The copy right of the authors.
Using a mouse brain ischemia model, we successfully demonstrated that intranasal administration of SA, dissolved in dimethysulfoxide not only reduced infarct size and protected blood brain barrier (BBB) integrity, but it also improved neurological outcome in a dose dependent manner [10]. Here we present some of the images that were not included in our publication. Figure 3 shows that the infarct size is reduced with increasing dose of SA, with the protective effect appearing to plateau at a dose between 25-50 µg/kg. Figure 4 shows that the BBB was well preserved in the presence of SA that was delivered by the intranasal route. These results are very critical since they demonstrate the effectiveness of intranasal SA administration, a very practical, fast and easy method to deliver medication to a patient in the field when IV access is not readily attainable. Since there is no need for extensive medical training to deliver a medication via the intranasal route, it can be administered by first responders. The dose in this study was only up to a maximum 50 µg/kg, which suggests strong potency of this compound. Intranasal delivery, which occurs via numerous paths that include entry to perineural spaces around, and endocytosis into, the olfactory nerve, as well as via the lymphatics and blood vessels of the nasal passage, offers the advantages of avoiding hepatic first pass metabolism and reducing potential off-target effects. This is because only a portion of the intranasal dose enters the systemic blood circulation via nasal capillaries. Since intranasal transport into the brain is not due primarily to passage into the blood, this offers the advantages of reduced systemic metabolism of the drug, and it offers the possibility of transport of drug to brain tissue that has experienced reduced, or absent blood flow due to cerebral ischemia.
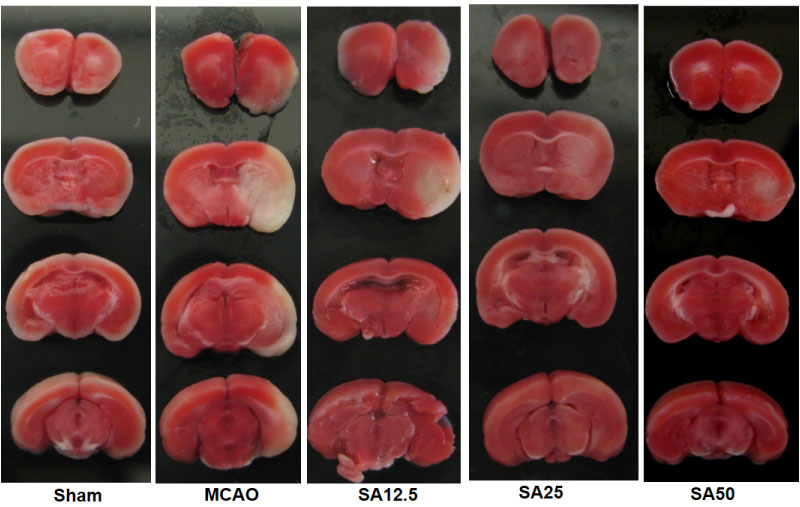
Figure 3: Brain slices of TTC (2,3,5-triphenyltetrazolium chloride) stain of mouse brain ischemia model in the presence and absence of intranasal SA. Infarction areas appear white due to reduced uptake of the dye. Infarcted area, especially in cortex decreased in SA treated animals at 2 mm, 4 mm, 6 mm and 8 mm from the anterior pole with dose of 12.5, 25 or 50 µg/kg. MCAO, middle cerebral artery occlusion; SA, Salvinorin A. Some of these images were presented in a previous publication (Chen C, et al. Crit Care Med. 2016;44(12):e1219-e25.). Please refer to this paper [10] for detailed experimental conditions.
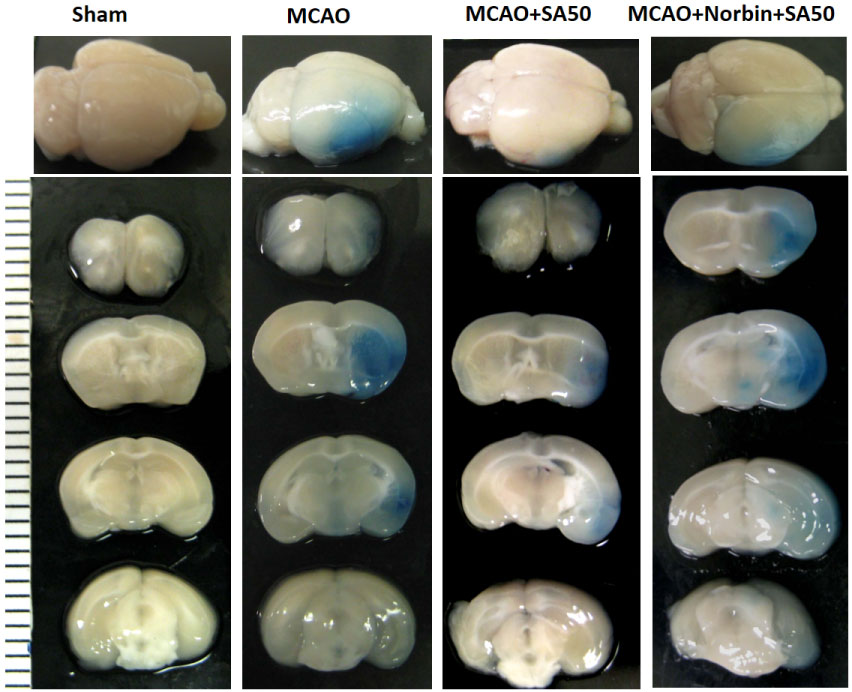
Figure 4: Representative images of Evans blue-stained brain sections of the mouse brain ischemia model in the presence and absence of intranasal SA. Evans blue staining was seen in the ischemic area in MCAO and in the KOR antagonist nor-BNI group. SA treatment without the KOR antagonist showed weak Evans blue staining in the striate area. nor-BNI given before the SA administration eliminated the protective effects of SA. SA, salvinorin A; KOR, kappa opioid receptor; nor-BNI, norbinaltorphimine. Part of the images were presented in the paper published in Critical Care Medicine (Chen C, et al. Crit Care Med. 2016;44(12):e1219-e25.), please refer the detailed experimental information in the paper [10].
The Effect on Subarachnoid Hemorrhage (SAH)
Patients who suffer a subarachnoid hemorrhage (SAH) are at risk of experiencing cerebral vascular spasm, a medical emergency which restricts blood circulation in the brain and can lead to delayed ischemic neurological deficit, the leading cause of morbidity and mortality in post-SAH patients [11]. In our recent studies, we demonstrated that administration of SA (10 µg/kg, intraperitoneal injection) at 24, 48, and 72 hours after SAH can prevent CVS, reduce neuronal apoptosis and injury, and improve neurological outcome in a rat SAH model [12,13]. This indicates the possibility that SA could be used in SAH to prevent vascular spasm and improve neurological outcome. Further studies are needed.
The Potential Barriers in Bring SA into Clinical Practice
SA has been consumed by human for centuries, it has some intrinsic side effects associated with its KOR agonism, the most significant one is the psychotic effect. SA is the only KOR agonist that has been consumed for recreational purposes due to its effect of euphoria rather than the dysphoria which is seen with most of classic KOR agonists. Although SA can generate hallucinatory effects, these effects are short-living due to the rapid hydrolysis of the SA molecule in the blood [14]. During brain ischemia, consciousness level of many patients is already impaired, with some stroke patients being in a comma status. Thus, any hallucinatory side effects may not even be perceived by patients when SA is administered. If neuroprotective effects of SA can be demonstrated in human subjects along with improved neurological outcomes in stroke patients, the benefits of using SA to rescue stroke patients certainly outweigh these non-life threatening short-lived risk, especially since there are no other medications available in stroke patients that offer widely-accepted neuroprotection.
Another potential barrier relates to the low water solubility of SA, resulting in the difficulty of SA delivery in forming suitable formulation for clinical usage. We have studied the chemical and physical properties of SA in the past [15] , and we believe that an intranasally deliverable system should and can be developed to treat stroke in a super acute setting. An intranasal delivery system would allow the medication to be delivered in a very timely manner [16].
In conclusion, the intrinsic characteristics of SA, including rapid onset of action, easy passage through the BBB via intranasal delivery, high potency in neurological protection with a clear mechanism of KOR agonism makes it a promising therapeutic agent for stroke. Further studies are warranted to develop this compound for clinical use.
Conflict of Interest
The authors are seeking potential clinical usage of salvinorin A for stroke
References
- Benjamin EJ, Virani SS, Callaway CW, Chamberlain AM, Chang AR, et al. (2018) Heart Disease and Stroke Statistics-2018 Update: A Report From the American Heart Association. Circulation 137: e67-e492.
- Feigin VL, Norrving B, Mensah GA (2017) Global Burden of Stroke. Circ Res 120: 439-448.
- Neuhaus AA, Couch Y, Hadley G, Buchan AM (2017) Neuroprotection in stroke: the importance of collaboration and reproducibility. Brain 140: 2079-2092.
- Moretti A, Ferrari F, Villa RF (2015) Neuroprotection for ischaemic stroke: current status and challenges. Pharmacol Ther 146: 23-34.
- Roth BL, Baner K, Westkaemper R, Siebert D, Rice KC, et al. (2002) Salvinorin A: a potent naturally occurring nonnitrogenous kappa opioid selective agonist. Proc Natl Acad Sci U S A 99: 11934-11939.
- Johnson MW, MacLean KA, Reissig CJ, Prisinzano TE, Griffiths RR (2011) Human psychopharmacology and dose-effects of salvinorin A, a kappa opioid agonist hallucinogen present in the plant Salvia divinorum. Drug Alcohol Depend 115: 150-155.
- Su D, Riley J, Kiessling WJ, Armstead WM, Liu R (2011) Salvinorin A Produces Cerebrovasodilation through Activation of Nitric Oxide Synthase, kappa Receptor, and Adenosine Triphosphate-sensitive Potassium Channel. Anesthesiology 114: 374-379.
- Wang Z, Ma N, Riley J, Armstead WM, Liu R (2012) Salvinorin A Administration after Global Cerebral Hypoxia/Ischemia Preserves Cerebrovascular Autoregulation via Kappa Opioid Receptor in Piglets PloS ONE 7: e41724.
- Su D, Riley J, Armstead WM, Liu R (2012) Salvinorin A pretreatment preserves cerebrovascular autoregulation after brain hypoxic/ischemic injury via extracellular signal-regulated kinase/mitogen-activated protein kinase in piglets. Anesth Analg 114: 200-204.
- Chen C, Xi C, Liang X, Ma J, Su D, et al. (2016) The role of kappa opioid receptor in brain ischemia. Crit Care Med 44: e1219-e1225.
- Dumont AS, Crowley RW, Monteith SJ, Ilodigwe D, Kassell NF, et al. (2010) Endovascular treatment or neurosurgical clipping of ruptured intracranial aneurysms: effect on angiographic vasospasm, delayed ischemic neurological deficit, cerebral infarction, and clinical outcome. Stroke 41: 2519-2524.
- Sun J, Yang X, Zhang Y, Zhang W, Lu J, et al. (2019) Salvinorin A attenuates early brain injury through PI3K/Akt pathway after subarachnoid hemorrhage in rat. Brain Res 1719: 64-70.
- Sun J, Zhang Y, Lu J, Zhang W, Yan J, et al. (2018) Salvinorin A ameliorates cerebral vasospasm through activation of endothelial nitric oxide synthase in a rat model of subarachnoid hemorrhage. Microcirculation 25: e12442.
- Hooker JM, Xu Y, Schiffer W, Shea C, Carter P, et al. (2008) Pharmacokinetics of the potent hallucinogen, salvinorin A in primates parallels the rapid onset and short duration of effects in humans. Neuroimage 41: 1044-1050.
- Orton E, Liu R (2014) Salvinorin A: A mini review of physical and chemical properties affecting its translation from research to clinical applications in humans. Transl Periop & Pain Med 1: 9-11.
- Chen C, Zhang M, Wu Y, Zhou C, Liu R (2019) Intranasal delivering method in the treatment of ischemic stroke. In: Chen J, Wang J, Wei L, Zhang J. Editors, Therapeutic Intranasal Delivery for Stroke and Neurological Disorders. Springer. pp. 75-89.